Fluorescence Microscopes
Elimination of Autofluorescence Blurring in Plants
-
Tags:
- Agriculture
What is autofluorescence?
Principle of autofluorescence
Autofluorescence is the natural emission of light called photoluminescence (PL) caused by certain cell structures and properties. When an object receives light, its molecules absorb photons (light), exciting the electrons. Photoluminescence is light emission that occurs as the electrons return to a lower energy state. Autofluorescence is present when cell organelles such as mitochondria and lysosomes, or components of the extracellular matrix such as collagen and elastin, or isoalloxazine ring flavin-containing compounds such as NADH and riboflavin naturally emit excitation light. Among plant cells, chlorophyll, a green pigment used in photosynthesis, and lignin that fills the cell walls are typical examples of elements that exhibit autofluorescence.
Wavelength of autofluorescence
In general, autofluorescence is observed when cell structures such as the above are excited with light that has a wavelength between 350 and 500 nm, and in return emit light with wavelengths as short as 350 to 550 nm.
For example, NADH, a typical flavin compound that emits autofluorescence, is excited by light in the 340-nm wavelength range and emits blue autofluorescence wavelengths around 460 nm. On the other hand, chlorophyll, which has the function of producing energy from light absorbed by the plant through photosynthesis, exhibits autofluorescence at a wavelength around 680 nm.
Understanding the wavelength of a substance’s excitation light along with its responsiveness thereto and the wavelength of its autofluorescence are key to a clear observation of the target substance and tissue.
Get detailed information on our products by downloading our catalog.
View Catalog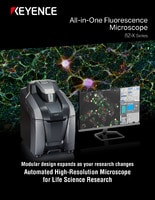
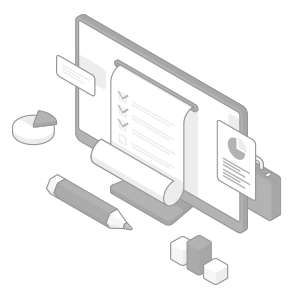
Challenges due to autofluorescence when observing plants and their solutions
Problems in fluorescence observation of plants
When observing multicellular organisms like plants, it is common to selectively label the target cells and structures using fluorescent proteins and enlarge the view for closer observation of these cells and tissues. However, if the signals of target cells and protein are weak under fluorescence microscopy when observing a plant, autofluorescence can be a factor preventing them from being distinguished. Particularly when observing plant cells, chlorophyll and other substances that exhibit autofluorescence are considered one of the main causes of fluorescence blurring.
Fluorescence blurring that occurs due to a plant’s autofluorescence makes observation of the target substance difficult, so the challenge is to counter the blurring in order to obtain clear images for easier observation and analysis.
Countering fluorescence blurring caused by autofluorescence
The use of filters is a common way to prevent the autofluorescence of chlorophyll from impeding observation of plant cells.
As mentioned earlier, the autofluorescence of chlorophyll is emitted in a wavelength range that peaks at 680 nm. Fluorescence observation can be achieved by using a fluorescence filter set suited for the wavelength band to eliminate light with a wavelength around 680 nm to ensure successful fluorescence observation free from effects of autofluorescence, such as fluorescence blurring.
If the wavelength of autofluorescence that is causing the fluorescence blurring cannot be identified, or it does not have unique wavelength characteristics, first you should find the wavelength range to cut out autofluorescence by testing different wavelengths using a standard fluorescence filter and then use an appropriate filter to facilitate the observation of the target substance.
Challenges and needs in plant observation
Eliminating autofluorescence-derived blurring using a filter requires time and effort to identify the autofluorescence wavelengths to be eliminated and the filters that correspond to them. When observing a plant’s intracellular structures, in some cases you might prepare specimens by dissecting organs and thinly slicing tissues into sections. Specimen preparation such as this requires advanced technical skills and experience. Additionally, the application of mechanical force on the plant while preparing sections poses a concern for causing unintended reactions.
Conventionally, it is also difficult to analyze and evaluate intrinsically three-dimensional structures from two-dimensional images of tissue sections. In short, the two major challenges and needs in the study of plants are to be able to selectively observe the target cells and tissues with clear, fluorescence blur-free images and to be able to accurately and efficiently observe three-dimensional structures.
We’re here to provide you with more details.
Reach out today!
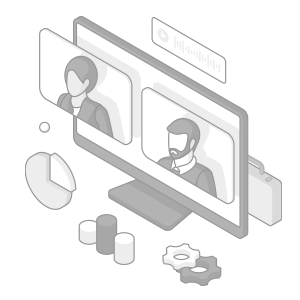
Capturing clear images without fluorescence blurring
Our latest fluorescence microscope can provide clear images with no fluorescence blurring caused by autofluorescence, and can also easily and quicky respond to different requirements and needs, such as three-dimensional observations. The following explains how this is achieved using an example of observation images of a potato plant as a case study.
Clear, fully focused images without fluorescence blurring
KEYENCE’s BZ-X800 All-in-One Fluorescence Microscope is equipped with the Sectioning function, which helps eliminate fluorescence blurring optically without the use of lasers and easily captures clear images.
The Sectioning function uses the optical sectioning algorithm* to eliminate fluorescence blurring, which leaves only clear fluorescence from the focal point position, allowing for quick and easy acquisition of clear images. Additionally, by capturing multiple images in the Z direction while using the Sectioning function, the BZ-X800 can accurately pick up only the fluorescence signals without being affected by fluorescence blurring at all heights over the entire depth of the specimen. Of those multiple images, the BZ-X800 singles out and combines only the areas that are at their sharpest focus, and uses them to create a fully focused image. This saves operators the time and labor needed to find the right filter in a conventional filter set that will eliminate the wavelength and brings them directly to a clear image for observation.
Normal observation
Z-stack & Full focus
Sectioning observation
Z-stack & Full focus
Objective lens: Plan Apochromat 40x
* The Optical Sectioning Algorithm uses structured illumination to section the areas of analyses (z-sectioning) when extracting only signals from the focused surfaces. Excitation light from a metal halide lamp that has a wide wavelength range from UV to near infrared is passed through an electronic projection element, which projects the light in a grid pattern onto the specimen (structured illumination). The grid is only projected onto the focused areas of the specimen. The BZ-X800 captures multiple images while moving the grid in the Z-direction, and by extracting only the areas onto which the grid is projected on from these images, prevents the effect of fluorescence blurring in the vertical direction. This algorithm automatically produces clear images selectively made up of only the signals of focused areas.
Original image
Grid projection
Grid moved in the Z-axis direction
Eliminate fluorescence blurring
3D image construction and flexible observation
Conventionally, it is difficult to analyze and evaluate three-dimensional tissue structures only using several two-dimensional images from multiple cross-section samples.
With the BZ-X800, you only need a single click to construct a 3D image from Z-stacked images captured at different points on the Z axis at a constant pitch. Additionally, users can rotate, zoom in or out, and observe cross-sections of the 3D image with just mouse actions while looking at the monitor.
3D images are all fully in focus regardless of angle, supporting accurate understanding of localized fluorescence signals however you move the mouse—you will never lose sight of your target cells or tissues.
Normal observation
Sectioning observation
Using the All-in-One Fluorescence Microscope BZ-X800
- The Sectioning function optically eliminates fluorescence blurring, allowing for the capture of clear images.
- The Z-stack function captures multiple continuous images on the Z axis at a specified pitch. From those images, the Full-focus function selects and combines only the areas that are at their sharpest focus. Together, these functions enable observation with images that are fully focused over the entire depth of the specimen.
- The BZ-X800 can easily construct accurate, high-precision 3D images based on Z-stack images acquired using the Sectioning function.
- Users can rotate, zoom in or out, and observe cross-sections of the 3D image as they wish just by moving the mouse. The BZ-X800 provides an accurate view of localized fluorescence signals from any angle.